3D-bioprinting improved artificial blood vessels
June 11, 2014
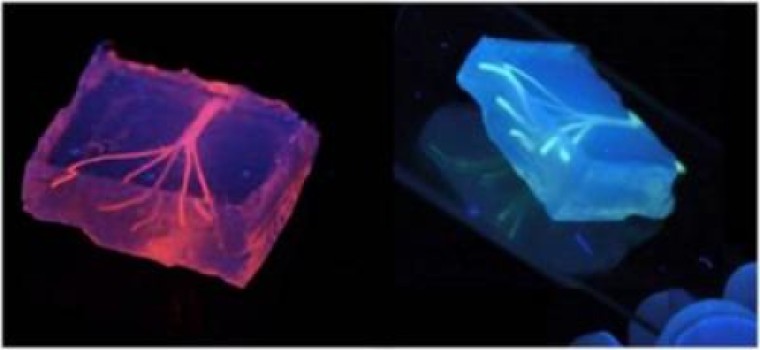
Artificial blood vessels are created using hydrogel constructs that combine advances in 3D bioprinting technology and biomaterials (credit: Khademhosseini Lab)
A Brigham and Women’s Hospital (BWH) team has created artificial blood vessels using a three-dimensional (3D) bioprinting technique.
The study is published online this month in Lab on a Chip.
“Engineers have made incredible strides in making complex artificial tissues such as those of the heart, liver and lungs,” said senior study author, Ali Khademhosseini, PhD, biomedical engineer, and director of the BWH Biomaterials Innovation Research Center. “However, creating artificial blood vessels remains a critical challenge in tissue engineering. We’ve attempted to address this challenge by offering a unique strategy for vascularization of hydrogel constructs that combine advances in 3D bioprinting technology and biomaterials.”
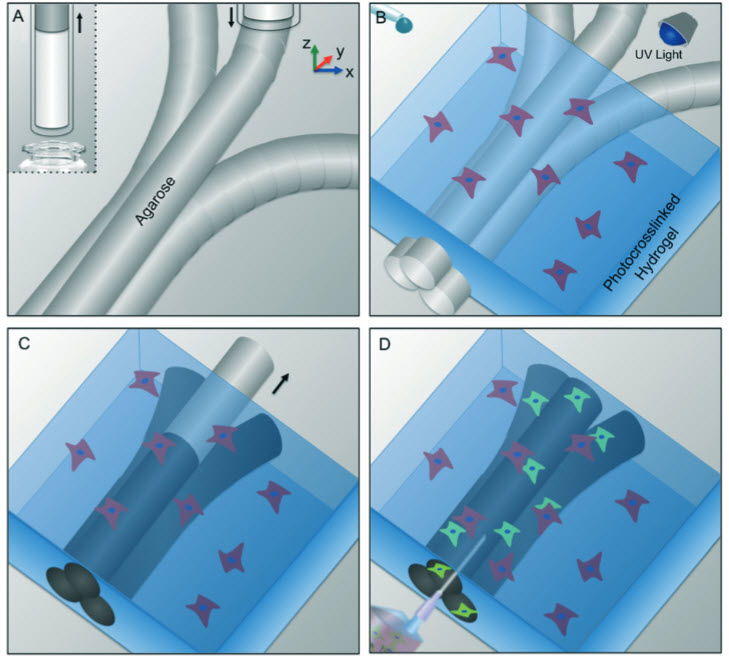
Schematic representation of bioprinting of agarose template fibers and subsequent formation of microchannels via template micromolding. a) A bioprinter equipped with a piston fitted inside a
glass capillary aspirates the agarose (inset). After gelation in 4° C, agarose fibers are bioprinted at predefined locations. b) A hydrogel precursor is casted over the bioprinted mold and photocrosslinked. c) The template is removed from the surrounding photocrosslinked gel. d) Fully perfusable microchannels are formed. (Credit: Luiz E. Bertassoni et al./Lab on a Chip)
The researchers first used a 3D bioprinter to make an agarose (naturally derived sugar-based molecule) fiber template to serve as the mold for the blood vessels.
They then covered the mold with a gelatin-like substance called hydrogel, forming a cast over the mold, which was then reinforced via photocrosslinks.
“Our approach involves the printing of agarose fibers that become the blood vessel channels. But what is unique about our approach is that the fiber templates we printed are strong enough that we can physically remove them to make the channels,” said Khademhosseini.
“This prevents having to dissolve these template layers, which may not be so good for the cells that are entrapped in the surrounding gel.”
Khademhosseini and his team were able to construct microchannel networks exhibiting various architectural features. They were also able to successfully embed these functional and perfusable microchannels inside a wide range of commonly used hydrogels, such as methacrylated gelatin or poly(ethylene glycol)-based hydrogels at different concentrations.
Khademhosseini said their fabricated vascular networks functioned to improve mass transport, cellular viability and cellular differentiation. Moreover, successful formation of endothelial monolayers within the fabricated channels was achieved.
“In the future, 3D printing technology may be used to develop transplantable tissues customized to each patient’s needs or be used outside the body to develop drugs that are safe and effective,” said Khademhosseini.
This research was supported by the National Institutes of Health (AR057837, DE021468, HL099073, AI081534, EB02597, GM095906), Presidential Early Career Award for Scientists and Engineers, Defense Threat Reduction Agency, and Australian Research Council.
Abstract of Lab on a Chip paper
Vascularization remains a critical challenge in tissue engineering. The development of vascular networks within densely populated and metabolically functional tissues facilitate transport of nutrients and removal of waste products, thus preserving cellular viability over a long period of time. Despite tremendous progress in fabricating complex tissue constructs in the past few years, approaches for controlled vascularization within hydrogel based engineered tissue constructs have remained limited. Here, we report a three dimensional (3D) micromolding technique utilizing bioprinted agarose template fibers to fabricate microchannel networks with various architectural features within photocrosslinkable hydrogel constructs. Using the proposed approach, we were able to successfully embed functional and perfusable microchannels inside methacrylated gelatin (GelMA), star poly(ethylene glycol-co-lactide) acrylate (SPELA), poly(ethylene glycol) dimethacrylate (PEGDMA) and poly(ethylene glycol) diacrylate (PEGDA) hydrogels at different concentrations. In particular, GelMA hydrogels were used as a model to demonstrate the functionality of the fabricated vascular networks in improving mass transport, cellular viability and differentiation within the cell-laden tissue constructs. In addition, successful formation of endothelial monolayers within the fabricated channels was confirmed. Overall, our proposed strategy represents an effective technique for vascularization of hydrogel constructs with useful applications in tissue engineering and organs on a chip.