An ultrafast 3-D imaging system to investigate traumatic brain injury
November 24, 2015
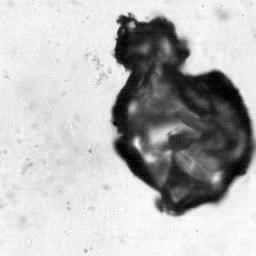
Still frame filmed at 200,000 frames/sec of a violently collapsing vapor bubble inside a brain-mimicking collagen gel (bubble size is approximately 100 microns). Inside the gel are thousands of brain cells (neurons). (credit: J. Estrada (Franck Lab)/Brown U)
Researchers at Brown University are using an ultrafast 3-D imaging system to investigate the effects of microcavitation bubbles on traumatic brain injury (TBI), experienced by some soldiers and football players.
In the fleeting moments after a liquid is subjected to a sudden change in pressure, microscopic bubbles rapidly form and collapse in a process known as cavitation.
In mechanical systems such as propellers, the resulting shock waves and jets can cause gradual wear, and in biological systems, they can shred and distort cells. In the human brain, this is believed to be a mechanistic cause of TBI, but the phenomenon has yet to be directly observed in brain tissue because the bubbles appear and disappear within microseconds.
To better understand the connection between microcavitation and traumatic brain injury, researchers at Brown University have developed a novel 3-D imaging system that allows them to film one million frames per second, with a single camera on a single microscope, and ultimately to explore mechanisms of damage to neurons in the laboratory.
Current 3-D image correlation methods typically involve making stereo projections of objects (projecting a sphere onto a plane) by capturing images with two or more cameras, but using multiple cameras carries a loss of spatial resolution. Instead, the researchers placed a diffraction grating in front of the imaging camera on the microscope. They then used a nanosecond-pulsed infrared laser to produce single cavitation bubbles within a model neural network made of collagen and biomimetic hydrogels embedded with neurons, thus simulating the action of a neural network subjected to a negative pressure surge.
It generates two perspectives of the object — as if you had two cameras — without sacrificing spatial resolution. Currently, they can resolve motion fields down to the 10–100 nanometer scale, but they are aiming for single-nanometer scale using super-resolution microscopy techniques in 3-D.
The researchers are presenting their recent findings at the American Physical Society (APS) Division of Fluid Dynamics (DFD) 68th meeting, Nov. 22–24 in Boston, Mass.
Abstract of Microcavitation as a Neuronal Damage Mechanism in Blast Traumatic Brain Injury
Traumatic brain injury (TBI), usually the result of impact or blast to the head, affects about 1.5 million Americans annually. Diffuse axonal injury, the hallmark feature of blunt TBI, has been investigated in direct mechanical loading conditions. However, recent evidence suggests inertial cavitation as a possible bTBI mechanism, particularly in the case of armed forces exposed to concussive blasts. Cavitation damage to free surfaces has been well-studied in the fi eld of fl uid dynamics, but bubble interactions within confi ned 3D environments have not been largely investigated. Cavitation occurs via a low-pressure region caused by pressure waves and is strongly dependent on local geometric and mechanical properties. The structural damage features as the result of cavitation – in particular at the cellular level – are incompletely understood, in part due to the rapid bubble formation and strain rates of up to ~105 –106 s– 1 . This project aims to characterize material damage in 2D and 3D environments and cell cultures by utilizing digital image correlation at a speed of up to ten 6 frames per second.