Decoding motion detection in the fly’s brain
July 13, 2010
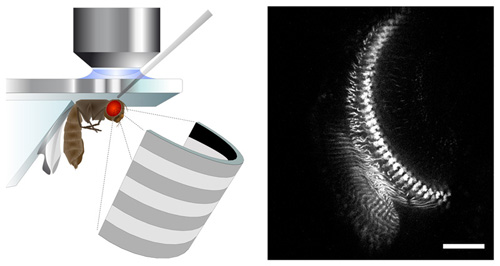
Neurobiologists use state-of-the-art methods to observe the activity of nerve cells while the fly sees moving stripe patterns on a LED screen (left). This technique allows for observing the response of single cells in the brain area that processes motion information (right, scale = 20 micrometer). (Max Planck Institute of Neurobiology)
Neurobiologists at the Max Planck Institute of Neurobiology have used state-of-the-art methods to decode the basics of motion detection in a fly’s brain.
While the number of nerve cells in the fly is comparatively small, they are highly specialized and process images with great precision while in flight. Flies can process a vast amount of information about motion and movement in their environment in real time. One sixth of a cubic millimeter of brain matter contains more than 100,000 nerve cells, each with multiple connections to its neighboring cells. Although it seems almost impossible to single out the reaction of a certain cell to any particular movement stimulus, this is precisely what neurobiologists at the Max Planck Institute of Neurobiology have now succeeded in doing.
Fluorescence molecules and state-of-the-art microscopes
“We had to find some way of observing the activity of these tiny nerve cells without electrodes,” as Dierk Reiff from the Max Planck Institute of Neurobiology explains. To overcome this hurdle, the scientists used the fruit fly Drosophila melanogaster and some of the most up-to-date genetic methods available. They succeeded in introducing the indicator molecule TN-XXL into individual nerve cells. By altering its fluorescent properties, TN-XXL indicates the activity of nerve cells.
To examine how the brains of fruit flies process motion, the neurobiologists presented the insects with moving stripe patterns on a light-diode screen. The nerve cells in the flies’ brains react to these LED light impulses by becoming active, thus causing the luminance of the indicator molecules to change. Although TN-XXL’s luminance changes are much higher than that of former indicator molecules, it took quite some time to capture this comparatively small amount of light and to separate it from the LED-light impulse. The solution was to synchronize a 2-photon-laser microscope with the LED-screen at a tolerance of merely a few microseconds. The TN-XXL signal could subsequently be separated from the LED-light and selectively measured using the 2-photon-microscope.
The cells behind the model
“After more than 50 years of trying, it is now technically possible to examine the cellular construction of the motion detector in the brain of the fly,” reports neurobiologist Alexander Borst. The scientists began by observing the activity of cells known as L2-cells which receive information from the photoreceptors of the eye. The photoreceptors react when the light intensity increases or decreases. The reaction of the L2-cells is similar in that part of the cell where the information from the photoreceptor is picked up. However, the neurobiologists discovered that the L2-cell transforms these data and in particular, that it relays information only about the reduction in light intensity to the following nerve cells. The latter then calculate the direction of motion and pass this information on to the flight control system. “This means that the information ‘light on’ is filtered out by the L2-cells,” says Reiff. “It also means, however, that another kind of cell must pass on the ‘light on’ command, since the fly reacts to both kinds of signals.”
The scientists next intend to examine, cell by cell, the motion detection circuitry in the fly brain to explain how it computes motion information at the cellular level.
Ref.: Visualizing retinotopic half-wave rectified input to the motion detection circuitry of Drosophila. Dierk F. Reiff, Johannes Plett, Marco Mank, Oliver Griesbeck, Alexander Borst. Nature Neuroscience, online publication from July 11, 2010
More info: Max Planck Society news