Microchip-like technology allows single-cell analysis
May 15, 2014
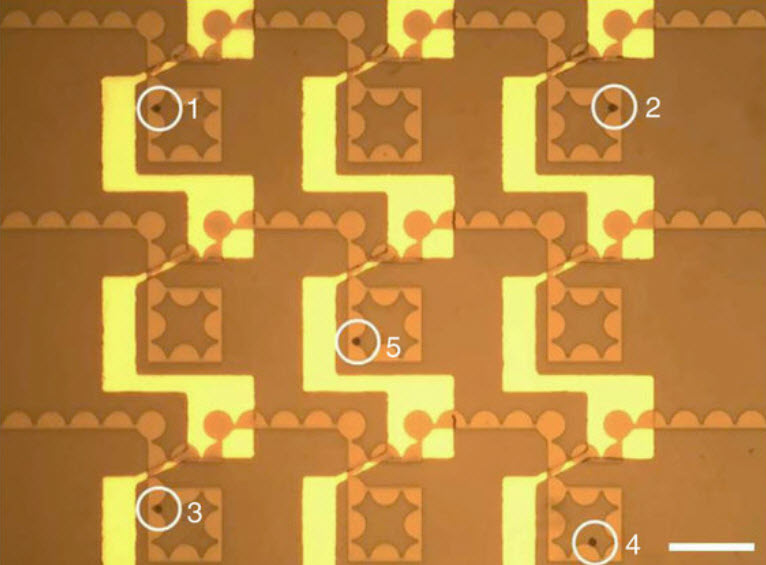
A 3-by-3 grid of compartments and switches, analogous to an integrated circuit (credit: Byeonghwa Lim et al./Nature Communications)
Using components similar those that control electrons in microchips, researchers at Duke University and Daegu Gyeongbuk Institute of Science and Technology (DGIST) in the Republic of Korea have designed a new device that can sort, store, and retrieve individual cells for study.
Similar to a random-access memory (RAM) chip, the device moves cells (rather than electrons) and could be scaled up to sort and store hundreds of thousands of individual living cells in a matter of minutes.
The researchers hope the cell-sorting system will revolutionize research by allowing fast, efficient control and separation of individual cells that could then be studied in vast numbers.
The study appears online May 14 in Nature Communications (open access).
Creating the equivalent of electronic circuits
“Most experiments grind up a bunch of cells and analyze genetic activity by averaging the population of an entire tissue rather than looking at the differences between single cells within that population,” said Benjamin Yellen, an associate professor of mechanical engineering and materials science at Duke’s Pratt School of Engineering.
“That’s like taking the eye color of everyone in a room and finding that the average color is grey, when not a single person in the room has grey eyes. You need to be able to study individual cells to understand and appreciate small but significant differences in a similar population.”
So Yellen and his collaborator, Cheol Gi Kim of DGIST, printed thin electromagnetic components like those found on microchips onto a slide. These patterns create magnetic tracks and physical elements analogous to switches, diodes, capacitors, and transistors that guide magnetic beads and single cells tagged with magnetic nanoparticles through a thin liquid film.
Like a series of small conveyer belts, localized rotating magnetic fields move the beads and cells along specific directions etched into a track, while built-in switches direct traffic to storage sites on the chip.
Creating an ‘integrated circuit’ for analysis of individual cells
In the study, the engineers demonstrate a 3-by-3 grid of storage compartments (analogous to random-access memory) overlaid with three horizontal row switches and three vertical column switches in multiplexed format (analogous to an integrated circuit). The demonstration shows how tagging cells with magnetic particles and directing them to different storage compartments could allow for the the cells to be separated, sorted, stored, studied, and retrieved.
Benjamin Yellen, associate professor of mechanical engineering and materials science, explains how his new cell sorting and storage system works. The technology works much like a computer chip, but it manipulates cells or magnetic particles instead of electrons.
In a random access memory chip, similar logic circuits manipulate electrons on a nanometer scale, controlling billions of compartments in a square inch. But cells are much larger than electrons; that limits the new devices to hundreds of thousands of storage spaces per square inch. Nonetheless, Yellen and Kim say that’s still small enough for their purposes.
“You need to analyze thousands of cells to get the statistics necessary to understand which genes are being turned on and off in response to pharmaceuticals or other stimuli,” said Yellen. “And if you’re looking for cells exhibiting rare behavior, which might be one cell out of a thousand, then you need arrays that can control hundreds of thousands of cells.”
As an example, Yellen points to cells afflicted by HIV or cancer. In both diseases, most afflicted cells are active and can be targeted by therapeutics. A few rare cells, however, remain dormant, biding their time and avoiding destruction before activating and bringing the disease out of remission. With the new technology, the researchers hope to watch millions of individual cells, pick out the few that become dormant, then quickly retrieve them and analyze their genetic activity.
“Maybe then we could find a way to target the dormant cells,” said Yellen.
Kim added, “Our technology can offer new tools to improve our basic understanding of cancer metastasis at the single cell level, how cancer cells respond to chemical and physical stimuli, and to test new concepts for gene delivery and metabolite transfer during cell division and growth.”
Scaling up
The researchers next plan to demonstrate a larger grid of 8-by-8 or 16-by-16 compartments with cells, and then to scale it up to hundreds of thousands of compartments. If successful, their technology would lend itself well to manufacturing, giving scientists around the world access to single-cell experimentation.
“Our idea is a simple one,” said Kim. “Because it is a system similar to electronics and is based on the same technology, it would be easy to fabricate. That makes the system relevant to commercialization.”
“There’s another technique paper we need to do as a follow-up before we get to actual biological applications,” added Yellen. “But they’re on their way.”
This research was supported by the National Science Foundation, the Research Triangle Materials Research Science and Engineering Center, and the National Research Foundation of Korea funded by the Ministry of Science, ICT & Future Planning.
Abstract of Nature Communications paper
The ability to manipulate small fluid droplets, colloidal particles and single cells with the precision and parallelization of modern-day computer hardware has profound applications for biochemical detection, gene sequencing, chemical synthesis and highly parallel analysis of single cells. Drawing inspiration from general circuit theory and magnetic bubble technology, here we demonstrate a class of integrated circuits for executing sequential and parallel, timed operations on an ensemble of single particles and cells. The integrated circuits are constructed from lithographically defined, overlaid patterns of magnetic film and current lines. The magnetic patterns passively control particles similar to electrical conductors, diodes and capacitors. The current lines actively switch particles between different tracks similar to gated electrical transistors. When combined into arrays and driven by a rotating magnetic field clock, these integrated circuits have general multiplexing properties and enable the precise control of magnetizable objects.