‘Superradiant’ discovery opens new path to superfast quantum computing
June 19, 2014
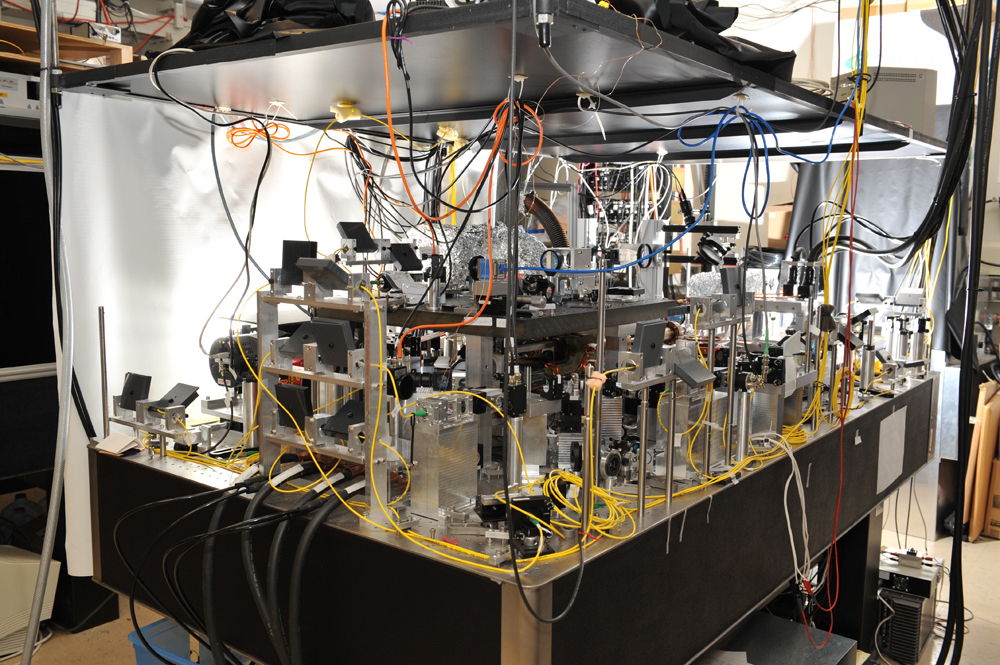
The atom trapping apparatus used to observe the physics described in the article (credit: Washington State University)
Washington State University researchers have used a super-cold cloud of atoms that behaves like a single atom, opening a new experimental path to potentially powerful quantum computing.
Physicist Peter Engels and his colleagues cooled about one million atoms of rubidium to 100 billionths of a degree above absolute zero.
There was no colder place in the universe, said Engels, unless someone was doing a similar experiment elsewhere on Earth or on another planet.
At that point, the cluster of atoms formed a Bose-Einstein condensate — a rare physical state predicted by Albert Einstein and Indian theorist Satyendra Nath Bose — after undergoing a phase change (similar to a gas becoming a liquid or a liquid becoming a solid).
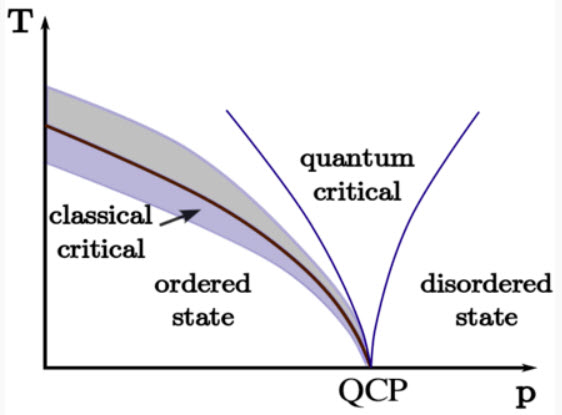
Phase transitions in condensed matter physics (T: temperature, p: phase, QCP: quantum critical point (credit: Wikimedia Commons)
Once the atoms acted in unison, they could be induced to exhibit coherent “superradiant” behavior, predicted by Princeton University physicist Robert Dicke in 1954, which describes the interactions between an ensemble of atoms and an optical field.
Engels explained the meaning of this discovery further in an email interview with KurzweilAI:
Our work provides a new handle on the Dicke model, a famous quantum mechanical problem that was first posed about 60 years ago. It concerns the interaction of an ensemble of atoms with light.
Robert Dicke realized that when the coupling between the light and the internal state of the atoms is sufficiently strong, the system can undergo a phase transition to a “superradiant” state. In practice, this phase transition turned out to be very difficult to observe because the required coupling strengths are not easily achieved in experiments (see, e.g., Nature 464, 1301–1306 (2010) for a first realization obtained by placing a Bose-Einstein condensate into a high finesse cavity).
In our experiment, we were able to realize this idea and test the predictions of the Dicke model, including the transition to a superradiant state. We started with a cloud of atoms held in an ultrahigh vacuum chamber. We laser-cooled the cloud to temperatures near absolute zero, where the cloud underwent a phase transition and formed a Bose-Einstein condensate, i.e., a macroscopic matterwave where nearly all atoms behave collectively and in unison.
Next, the atoms were placed in a suitably tailored laser field that facilitated the coupling between the internal state of the atoms and their motion. Upon changing the laser parameters, the transition to the superradiant state was observed. With this, we now have a system available with which the Dicke model can readily be realized and tested.
This provides a new avenue for the study of further Dicke model-related phenomena, including quantum entanglement and quantum squeezed states, with possible applications for a next generation of atomtronic devices, quantum information storage/transmission, quantum computations and quantum precision measurements.
What our colleague Chuanwei Zhang realized is, if you replaced the light with the motion of the particles, you got exactly the same physics. Moreover, it’s easier to observe. So while their cloud of atoms measures less than half a millimeter across, it is large enough to be photographed and measured. This gives experimenters a key tool for testing assumptions and changes in the atomic realm of quantum physics.
Engels’ findings appear in the journal Nature Communications. Funders include the National Science Foundation, the Army Research Office DARPA.
Abstract of Nature Communications paper
Spin-orbit-coupled Bose–Einstein condensates (BECs) provide a powerful tool to investigate interesting gauge field-related phenomena. Here we study the ground state properties of such a system and show that it can be mapped to the well-known Dicke model in quantum optics, which describes the interactions between an ensemble of atoms and an optical field. A central prediction of the Dicke model is a quantum phase transition between a superradiant phase and a normal phase. We detect this transition in a spin-orbit-coupled BEC by measuring various physical quantities across the phase transition. These quantities include the spin polarization, the relative occupation of the nearly degenerate single-particle states, the quantity analogous to the photon field occupation and the period of a collective oscillation (quadrupole mode). The applicability of the Dicke model to spin-orbit-coupled BECs may lead to interesting applications in quantum optics and quantum information science.