Ultra-fast ‘phase-change materials’ could lead to 1,000-times-faster computers
September 25, 2014
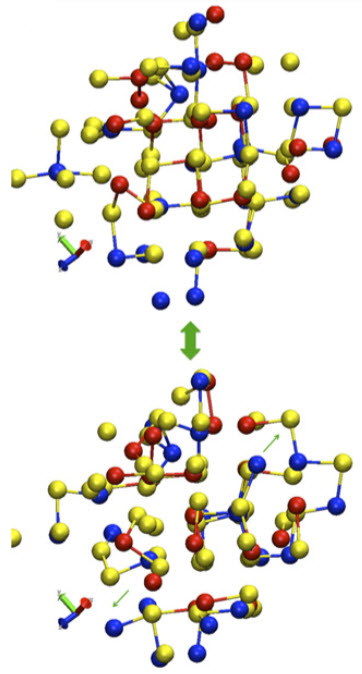
Solid (top) to liquid (bottom) transition in Germanium-tin antimony-telluride (Ge–Sb-Te, or GST) alloy model (Ge: blue, Sb: red, Te: yellow) (credit: Desmond Loke et al./PNAS)
Replacing silicon, new ultra-fast “phase-change materials” (PCMs) that could eventually enable processing speeds 500 to 1,000 times faster than the average laptop computer today — while using less energy — have been modeled and tested by researchers from the University of Cambridge, the Singapore A*STAR Data-Storage Institute, and the Singapore University of Technology and Design.
PCMs are capable of reversibly switching between two structural phases with different electrical states — one crystalline and conducting and the other glassy and insulating — in billionths of a second, increasing the number of calculations per second.
Also, logic operations and memory are co-located, rather than separated, as they are in silicon-based computers (causing interconnect delays and slowing down computation speed), and PCM devices can function down to about two nanometers (compared to the current smallest logic and memory devices based on silicon, which are about 20 nanometers in size). The researchers have also demonstrated that multiple parallel calculations are possible for PCM logic/memory devices.
Achieving record switching speed
The researchers used a new type of PCM based on a specific chalcogenide glass material that goes further: it can be melted and recrystallized in as little as 900 picoseconds (trillionths of a second) using appropriate voltage pulses.
PCM devices recently demonstrated to perform in-memory logic do have shortcomings: they do not perform calculations at the same speeds as silicon, and they exhibit a lack of stability in the starting amorphous phase.
However, the Cambridge and Singapore researchers found that, by performing the logic-operation process in reverse — starting from the crystalline phase and then melting the PCMs in the cells to perform the logic operations — the materials are both much more stable and capable of performing operations much faster.
The results are published in the journal Proceedings of the National Academy of Sciences.
Limits to miniaturization and speed
The calculations performed by most computers, mobile phones, and tablets are carried out by silicon-based logic devices. The solid-state memory used to store the results of such calculations is also silicon-based. “However, as demand for faster computers continues to increase, we are rapidly reaching the limits of silicon’s capabilities,” said Professor Stephen Elliott of Cambridge’s Department of Chemistry, who led the research.
The primary method of increasing the power of computers has been to increase the number of logic devices that they contain by progressively reducing the size of the devices; but physical limitations for current device architectures mean this is quickly becoming nearly impossible to continue.
In addition, logic and memory devices are currently constructed in layers. As the devices are made ever smaller (to increase device density), eventually the gaps between the layers will get so small that electrons that are stored in certain regions of flash non-volatile memory devices will be able to tunnel out of the device, resulting in data loss.
New processor and memory-device materials
First developed in the 1960s, PCMs were originally used in optical-memory devices, such as re-writable DVDs. Now, they are starting to be used for electronic-memory applications and are beginning to replace silicon-based flash memory in some makes of smartphones.
The intrinsic switching, or crystallization, speed of existing PCMs is about ten nanoseconds, making them suitable for replacing flash memory. By increasing speeds even further, to less than one nanosecond (as demonstrated by the Cambridge and Singapore researchers in 2012), they could one day replace computer dynamic random-access memory (DRAM), which needs to be continually refreshed, by a non-volatile PCM replacement.
“Eventually, what we really want to do is to replace both DRAM and logic processors in computers by new PCM-based non-volatile devices,” said Professor Elliott. “But for that, we need switching speeds approaching one nanosecond. Currently, refreshing of DRAM leaks a huge amount of energy globally, which is costly, both financially and environmentally. Faster PCM switching times would greatly reduce this, resulting in computers which are not just faster, but also much ‘greener’.”
The research was part-funded by the UK Engineering and Physical Sciences Research Council (EPSRC).
Abstract of Proceedings of the National Academy of Sciences paper
The ultrahigh demand for faster computers is currently tackled by traditional methods such as size scaling (for increasing the number of devices), but this is rapidly becoming almost impossible, due to physical and lithographic limitations. To boost the speed of computers without increasing the number of logic devices, one of the most feasible solutions is to increase the number of operations performed by a device, which is largely impossible to achieve using current silicon-based logic devices. Multiple operations in phase-change–based logic devices have been achieved using crystallization; however, they can achieve mostly speeds of several hundreds of nanoseconds. A difficulty also arises from the trade-off between the speed of crystallization and long-term stability of the amorphous phase. We here instead control the process of melting through premelting disordering effects, while maintaining the superior advantage of phase-change–based logic devices over silicon-based logic devices. A melting speed of just 900 ps was achieved to perform multiple Boolean algebraic operations (e.g., NOR and NOT). Ab initio molecular-dynamics simulations and in situ electrical characterization revealed the origin (i.e., bond buckling of atoms) and kinetics (e.g., discontinuouslike behavior) of melting through premelting disordering, which were key to increasing the melting speeds. By a subtle investigation of the well-characterized phase-transition behavior, this simple method provides an elegant solution to boost significantly the speed of phase-change–based in-memory logic devices, thus paving the way for achieving computers that can perform computations approaching terahertz processing rates.